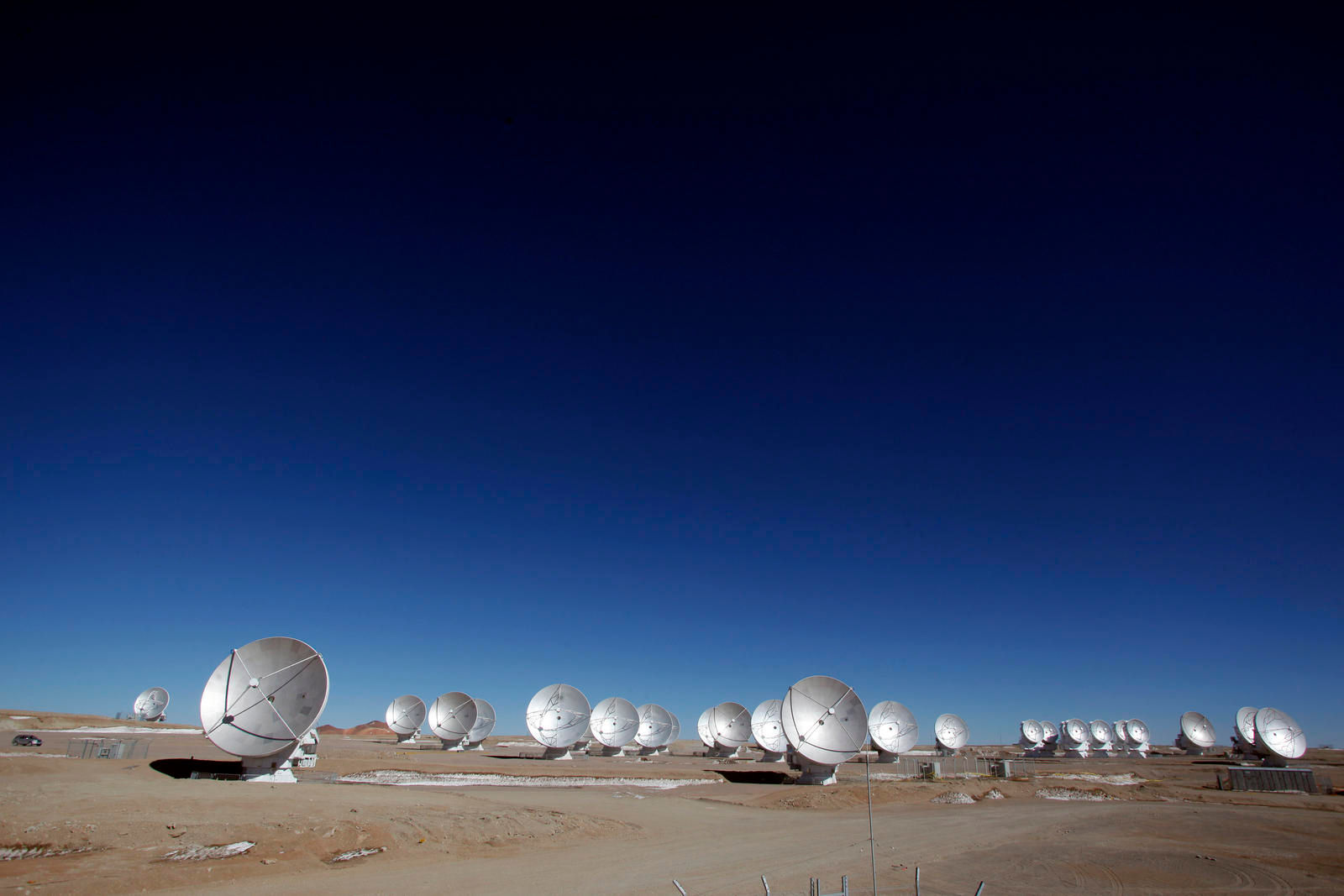
Ongoing Astronomical Research
Introduction
Understanding how galaxies form and evolve as a function of cosmological time (z, redshift) is a key goal in modern astrophysics. Standard theoretical models address this problem in a framework assuming a Lambda-CDM cosmology (Spergel et al. 2003), where the hierarchical gravitational grow of dark matter haloes trace the large-scale structure of the observed baryonic matter (Springel et al. 2006). This framework is governed by an easy set of differential equations which can be computationally solved by the powerful modern computers (Springel et al. 2005; Boylan-Kolchin et al. 2009; Klypin et al. 2011; Springel 2012). Nevertheless, at galactic-scales the evolution is driven by dissipative non-linear processes which are far more complex than what the theory could predict. Therefore observations of different kind of galaxies, and clusters of galaxies, at all redshifts become an essential ingredient to feed semi-analytical models of galaxy formation and evolution.
Throughout cosmic time, the hierarchical co-evolution of dark matter haloes and baryonic matter is intimately linked to the star formation activity, i.e. to the way in which the gas is gravitationally collapsed to form stars (see annex Figure 1). Living within these dark matter haloes, galaxies are fed continuously by gas from the intergalactic medium through gas cooling, cold-flows and minor/major mergers. The new born stars ionise the surrounding medium dramatically changing the properties of the Inter-Stellar Medium (ISM) of the galaxy. The most massive stars then explode as supernovae, feeding back vast amount of energy into the ISM, while at the same they enrich the ISM with heavy metals. These metals change the process in which light is absorbed and reprocessed. The energy injected by the star-formation activity is recycled by the surrounding media, an effect referred as feedback and which is a critical ingredient on galaxy formation and evolution models.
The rate at which galaxies form new stars (the Star Formation Rate; SFR) is found to be strongly correlated with the mass already in stars (the stellar mass; Mstar). This correlation defines a typical average value for the specific SFR (sSFR = SFR/Mstar) at a given mass and redshift bin (e.g. Noeske et al. 2007, Elbaz et al. 2007, Daddi et al. 2007, Pannella et al. 2009). In other words, more violent star formation is seen in more massive galaxies. Some rare galaxies display an excess in sSFR, thought to be due to a higher star-formation efficiency in compact nuclear star-forming regions – probably triggered by the merger of two or more galaxies (e.g. Daddi et al. 2010).
The Super-Massive Black Hole (SMBH) at the centre of a massive galaxy is spatially tiny (relative to the galaxy-scale), but it plays a key role in the evolution of galaxies. It is thought that the intimate relation between SMBHs and galaxies is a product of a cosmic cycle driven by major mergers. When gas-rich galaxies collide, they trigger a gas inflow (thanks to the loss of angular momentum) which fuels a violent nuclear starburst, while simultaneously producing a feedback-regulated SMBH growth. Eventually, the system should reveal a bright quasar (an extremely luminous accretion disk around the SMBH), after which exhaustion and violent relaxation transform the remnant into a red elliptical galaxy (e.g. Hopkins et al. 2006). Approximately 10% of all powerful AGNs expel relativistic particles from the vicinity of the SMBH via collimated jets, also feeding back some of the energy into the ISM of the galaxy. This feedback mechanism has become an essential mechanism to accommodate semi-analytical modelling of galaxy evolution in a Lambda-CDM framework.
Observationally speaking, panchromatic long exposure imaging (‘deep-field’ surveys) – apart from looking back in time out to the epoch were the first galaxies were formed – have provided an unprecedented view to the evolution of galaxies throughout cosmic time. For example, Hubble Space Telescope (HST) images taken in the visible/near-IR regime of the electromagnetic spectrum have enabled us to understand the built up of stars through cosmic times to unprecedented detail. These magnificent HST pictures are, however, affected by extinction (scattering by gas and absorption by dust) along the line of sight, i.e. the observed light is a small fraction of the total emitted by the galaxies (e.g. Ibar et al. 2013). A few years ago, studies of the dust and molecular gas content in galaxies (the raw fuel from which stars form) have been fundamentally limited due to the lack of sensitivity of previous astronomical facilities working at radio and sub-millimetre frequencies -- the regime at which it is possible to detect the gas emission coming from large cosmological distances. The advent of the Atacama Large Millimeter/sub-millimeter Array (ALMA), in Chilean territory, promises to change these limitations and revolutionize our understanding of the formation of galaxies. ALMA has become the most sensitive sub-millimeter telescope, capable to detect dust and gas from galaxies out a few hundred million years after the Big Bang (cosmic redshift z~8-10). ALMA and upcoming future gigantic telescopes as the Giant Magellan Telescope (GMT) and the European-Extremely Large Telescope (E-ELT), will be key to complete the puzzle to understand the cosmic evolution of galaxies, making a breakthrough in astrophysics which will not only catch the attention of the scientists but also the general public.
Detailed ISM studies of gravitational lenses at high-redshift
One of the most interesting results obtained from the Herschel Space Observatory is the clean and easy detection of a significant number of gravitationally lensed galaxies. Large-area surveys, such as H-ATLAS and HerMES, have sufficient areal coverage to detect the very brightest SMGs in the Universe, among them highly- and moderately-magnified `typical' star-forming galaxies at high redshift (e.g. Blain 1996). For instance, Negrello et al. (2010) detected numerous ultra-bright far-IR sources in the early Herschel data which comprise a mixture of strongly lensed SMGs, plus `contaminants' -- nearby (z< 0.3) spirals and blazars (easily removed via optical SDSS and radio FIRST data) -- and demonstrated via high-resolution interferometric SMA-880microns imaging the multiple-component nature of the lenses. Previously, relatively tiny surveys for SMGs uncovered no more than a handful of sufficiently bright SMGs to allow high-SNR and high-resolution investigations with current telescopes. Using gravity as a `cosmic telescope' is particularly useful in the sub-mm waveband as it alleviates photon starvation, lowers the flux limit at which confusion dominates and facilitates the identification of counterparts. Indeed, gravitational lensing has allowed very detailed studies of those `typical' high-redshift star-forming galaxies that dominate the cosmic far-IR background yet are lost beneath the confusion limit of Herschel (e.g. Ivison et al. 2010; Lupu et al. 2011; Valtchanov et al. 2011). Sub-mm spectroscopy has confirmed that these are massive, gas-rich, star-forming galaxies at z~1-4, and revealed the ionising mechanisms (star-formation or AGN) affecting their inter-stellar medium (ISM). At this level of sophistication (and statistics) the analysis of the ISM at z > 2 begins to be comparable to that of z~0 galaxies -- a striking demonstration of the opportunities which gravitational lensing provides to aid our understanding of the processes which drive galaxy formation and evolution.
I lead the follow-up campaign using high-resolution radio observations with the EVN and eMERLIN telescopes of some of these gravitationally lensed galaxies. I have demonstrated milli-arcsec detections of compact AGNs in some of these objects, while also putting tight constraints on lens modellings. During the next years, I plan to continue this high-resolution campaign using the ALMA observatory.
The [CII] and the CO emission explored in a multi-wavelength fashion
At far-IR wavelengths, the most prominent emissionline is [CII]-157.74 μm, which carries 0.1 − 1% of the bolometric far-IR power emitted by star-forming galaxies (Stacey et al. 1991,2010), with [CII] fluxes which are typically more than a thousand times stronger than CO(1–0) at 115GHz. Its low ionisation potential, 11.26 eV, makes it a key ingredient for cooling the warm and diffuse media and convert it into cold and dense clouds, which will be then collapse to form stars (Dalgarno & McCray 1972). This fine structure line is an excellent tracer of the different stages of evolution of the ISM as it can be excited by collisions with electrons, HI and H2 in the warm ionised medium, the warm and cold diffuse medium and warm and dense molecular gas. Its intensity is sensitive to the column density, the volume density and the kinetic temperature of the ISM (Pineda et al. 2013). Therefore, the [CII] line is a powerful tool to trace the diffuse neutral gas, but also of ionised HII regions including their surrounding PDRs affected by the far-ultraviolet (-UV) radiation fields from the central hot, young stars.
The bulk of the molecular gas mass of the ISM is comprised of H2 (molecular Hydrogen) -- the reservoir for future star-formation. Nevertheless, this molecule lacks of a strong net dipole moment, implying direct observations are extremely dicult (e.g. Papadopoulos & Seaquist 1999; Bothwell et al.2012). Fortunately, the molecular gas is not pure H2. 12CO (the second most abundant molecule in the ISM) has a ground rotational transition (J=1-0 at rest 115.271 GHz) which is low enough to be easily excited, even deep inside cold molecular clouds (A_V > 2-5) where it can be easily thermalised by collisions with H2 at relatively low n(H2)~300 cm^-3 densities. 12CO(1-0) has thus become the workhorse tracer ofthe bulk distribution of H2 in our Galaxy and beyond (Bolatto et al. 2013).
Combining two of the major extragalactic surveys, H-ATLAS and GAMA, I am performing a detailed study of the parameters controlling the [CII] and CO emission, by combining Herschel photometry, PACS spectroscopy and new ALMA Band-3 data (over 20hrs in Cycle-1 & 2). High-quality spectra and far-IR photomery is providing key insights for the mechanisms controlling the so-called [CII]-deficit.
Star-formation and AGN activity revealed via submm/radio interferometry
For many years, studies of high-z star-forming galaxies have been limited to low resolution imaging, i.e. providing global properties and unable to describe the intricate ongoing physics. Thanks to the advent of sub-arcsecond and sensitive far-IR/radio imaging, we can now resolve and potentially isolate the physical mechanisms dominating these galaxies. These new available capabilities will allow to tackle questions such as, what is triggering their star-formation activity? are they massive mergers or disk-like galaxies? what is the role of AGN on their evolution?.
The advent of the Atacama Large Millimeter/sub-millimeter Array (ALMA) promises to revolutionize our understanding of the formation of galaxies. ALMA is sensitive to the sub-millimeter part of the spectrum where galaxies are expected to be easily detectable out to the epoch of reionization -- a few hundred million years after the Big Bang (cosmic redshift z~8). Thus, ultra deep spectroscopic (and continuum) observations with ALMA can provide unbiased measurements of the molecular gas (and dust), and thereby help us to complete the puzzle to understand the cosmic evolution of galaxies. Since such ultra-deep millimeter spectroscopy of the Universe has never been performed, such observations will open up new windows for serendipitous discoveries (i.e. gas-rich dark galaxies) and will bring up a new view of the Universe, hidden to our eyes.
Nowadays, I am involved in a four recently awarded ALMA ’blind deep-field’ projects (adding up yo 80hrs in Cycle-1 & 2 -- data not yet delivered) to study galaxy formation and evolution, including continuum imaging of the HUDF and Frontier Fields, and also an experiment performing dual-band-scanning of the HUDF.
In the past, I have been responsible for cataloguing and imaging several deep radio legacy fields, including GOODS-N (Morrison et al. 2010), Lockman Hole (Ibar et al. 2009), SXDF (Arumugam et al., in prep), and ECDFS (Biggs et al. 2011), while also being a member of the EMU survey (Norris et al. 2011) -- a future all sky survey using the Australian SKA Pathfinder (ASKAP).
Star-forming Halpha emitters at high redshift
Historically, the classical star-formation rate (SFR) indicator has been the Halpha (lambda_rest=656.3nm) luminosity -- a well-calibrated indicator of instantaneous emission from massive, young stars (<20Myr and >8M_o; e.g. Kennicutt 1998). To extrapolate the radiation fields of O and B stars to the total SFR of a galaxy requires careful consideration of the initial mass function of their stellar population, and the amount of extinction suffered by their starlight. The main complication is the sensitivity of Halpha flux to extinction. The observed line fluxes represent only a fraction of the intrinsic emission, with typical values of Halpha extinction (A_Halpha, where L(Halpha)_intrinsic=L(Halpha)_observed x 10^(0.4 x A_Halpha)) found to be ~0.8-1.1mag (e.g. Garn & Best 2010). In general, when the level of extinction is low or moderate, A_Halpha ~< 3mag, the difference between the observed Balmer decrement (Halpha/Hbeta) and the theoretical expectation (2.86 for Case B recombination; see Kennicutt 1998) can determine the amount of extinction.
Those ultra-violet/optical photons that are absorbed by the surrounding media are re-emitted in the far-IR waveband, meaning that far-IR can also be used as a tracer of the SFR and/or the amount of extinction in the system. If all the starlight is absorbed then the system works as a calorimeter and the far-IR becomes the ideal tracer of SFR. Recently, Kennicutt et al. (2009) parameterised the SFR as a linear combination of the observed Halpha and bolometric far-IR luminosities -- an estimator which addresses the contributions from unobscured and obscured star-formation.
Garn & Best (2010) showed that one of the strongest parameters controlling the extinction in galaxies is stellar mass (M_star), suggesting that the level of extinction produced by the material in and surrounding their star-forming regions increases as galaxies built up their M_star. Interestingly, metallicity was found to be an inferior tracer of extinction. On the other hand, previous studies have shown that M_star plays a key role in controlling the amount of star formation (e.g. Elbaz et al. 2007, Daddi et al. 2007). For `star-forming galaxies', the SFR is found to be linearly correlated to M_star and defines a typical value for the specific SFR (sSFR=SFR/M_star), where more violent star formation is seen in more massive galaxies. Controversially using Herschel data, Elbaz et al. (2011) propose the existence of two modes of star formation: `normal' galaxies which lie in a well-defined parameter space (the `main sequence') defined in a plot of sSFR versus redshift, and `starburst' galaxies which present an excess in sSFR related to an increment of efficiency in compact star-forming regions probably triggered by the merger of two or more galaxies.
I have an ongoing campaign with ALMA (over 20hrs in Cycle-1 & 2) for characterising the spatial distribution of the dust at matched resolution to new SINFONI AO-aided imaging (over 40hrs of data to date). The sample is extracted from the HiZELS survey at z=0.84, 1.47 and 2.23. ALMA detections in CO are also available for some of these galaxies, allowing derivation of the reservoir of star-formation.